ABOUT
The project
WISE sets a new frontier for product complexity by shifting the focus from geometry to functionality, introducing features such as self-healing, triggered biomolecule diffusion, and smart repairing. This disruption redefines current design methodology, leveraging advanced AI-aided engineering and multi-scale, multi-process manufacturing to achieve unprecedented complexity. WISE has the potential to drive a discontinuity in the growth of the European industry by convincingly demonstrating an innovative, competitive, and scalable manufacturing concept based on a single machine that integrates macroscale processing (i.e., DED via hybrid blue-IR lasers) and micro-nano scale processing (i.e., fs and ns laser ablation, 2PP, DALP).
WISE will deliver a TRL7 multi-process machine integrating high-precision, multi-scale techniques for producing complex multifunctional products. The project will demonstrate the machine’s performance, flexibility, and precision by producing three complex hybrid products in MedTech, Aerospace, and Power generation sectors. The project builds upon TRL5-validated results from previous H2020 projects, which have brought significant innovations in the field of advanced manufacturing and enabling technologies.
The work plan includes a final demonstration to validate the solution through the production of complex components based on end-user requirements. Upon completion, WISE will be further developed to reach TRL9 within 24 months, leading to its commercial launch as a multi-process manufacturing station.
The WISE consortium is composed of 16 partners, including 8 SMEs, 4 LE, and 4 RTD. Their expertise covers mechatronics, intelligent nanostructured components, laser-material interaction, high-precision machines and tools. The project partners form a cluster of European countries playing crucial roles in the production of functionalized products with smart functionalities, adaptive photonic solutions, and advanced mechatronic systems.
The challenges
What if complexity would no longer be what we’re used to interpret in manufacturing, i.e. geometrical. Smart functionalities within the material are what we consider as complexity in products. Self-Healing Materials, Triggered Biomolecules Diffusion, Smart Repairing Processes are our target functionalities; materials that can feel history and the context, being also sensors, and set in place a strategy to optimize their behaviour.
Additive Manufacturing (AM) established a new paradigm to design products, layer-wise. And it is exactly what enables the opportunity to incorporate complex nano-scale molecules into a macro layered structure.
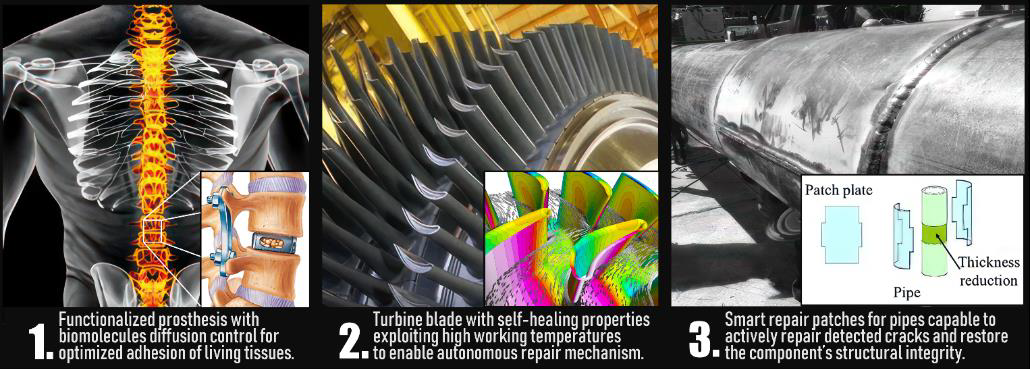
In the current manufacturing landscape, realizing such advanced functionalities is hindered by the limitations of individual process technologies, regardless of how novel they may be. Product designs must conform to the constraints of each technology, which subsequently restricts the potential for redesign and the incorporation of new functionalities. WISE intends to revolutionize this paradigm, by integrating multiple functions, processes, and scales into a single lifecycle design and into a single manufacturing machine (Fig. 1). This approach will break down these barriers, unlocking the full potential of advanced manufacturing for complex, multifunctional products.
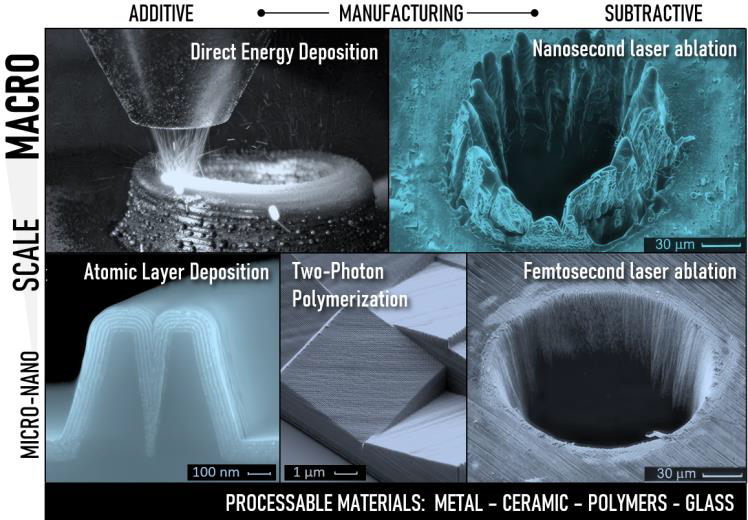
complex product from macro- to nanoscale
WISE can trigger a discontinuity in the change and growth of European industry, through transitioning products and materials to a maturity stage where smart functionalities would become industrially-grade; the convincing demonstration of an innovative, competitive and scalable manufacturing concept to be integrated at large-scale in the European factory shop-floors based on asingle- machine integrating macroscale processing (i.e. DED via hybrid blue-IR lasers) and micro-nano scale processing (i.e. fs and ns
laser ablation, 2PP, DALP), operating on a single working volume; disrupting the current lifetime prediction models for structural
high-value added products by relying upon an advanced AI-based platform that leverages manufacturing quality measurements as well as operative data (Fig. 2).
WISE will deliver knowledge, processes, and equipment to enable the cost-effective, high-throughput, high-quality production of new complex hybrid products, with enhanced functionalities.
Three novel functionalities will be demonstrated:
F1 – Triggered Biomolecules Diffusion (TBioD). Functionalization of spinal cage prosthesis with biocompatible nanocoating enables the diffusion of therapeutic organic molecules on the surface. The prosthesis core consists of a biocompatible metal alloy (e.g., Ti-6Al-4V), functionalized with surface nanostructures to promote TBioD (Fig. 3). The nanostructures in contact with bone tissues are coated with a biocompatible polymeric layer, fostering photoreactive biomolecule adhesion (such as BMP-complexes) to ensure enhanced bioactive behaviour and boost bone fusion. Surfaces not responsible for osseointegration are coated with an ultra-thin ceramic layer to improve biocompatibility and increase wear/corrosion resistance. Biomolecule transport is activated by local electromagnetic fields (ECs) induced by surface plasmon resonance (LSPR) and surface plasmon polaritons (SPPs). The functionalized spinal cage features a consolidated design that shortens surgical operation time by one-third and raises the surgery’s success rate. Patients experience reduced convalescence and post-treatment time by decreasing the risk of complications during the healing process (e.g., aseptic loosening and implant mobilization).
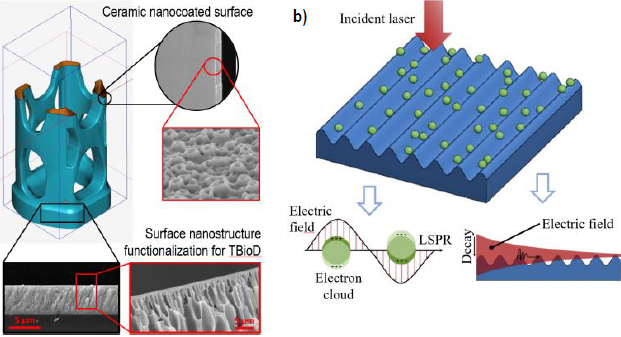
(b) Laser-induced TBioD
F2 – Self-Healing (SH). Smart Complex Concentrated Materials (CCMs) with self-healing mechanisms for severe working environments. New turbine blades, designed for fatigue resistance, utilize advanced functional metal composites with High-Entropy CCMs for self-repair in harsh conditions, including corrosive environments and high temperatures (Fig. 4). The Ni-based superalloy core is coated with a functionally graded Complex Concentrated Alloys (CCAs) layer, ensuring solid phase stability up to 700°C. A Complex Concentrated Oxides (CCOs) skin is applied for thermal insulation, maintaining mechanical performance and reducing wear and corrosion up to 1500°C. High-Entropy and severe lattice distortion effects in CCMs promote solid-state phase stability at high temperatures, enabling atomic element diffusion and redistribution for autonomous repair under external heat input.
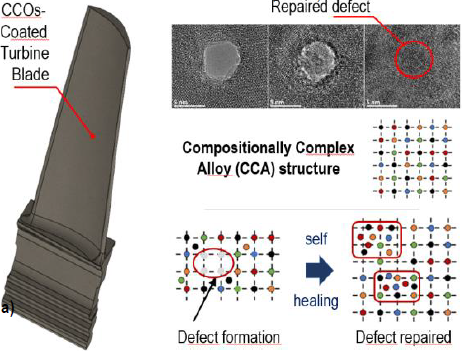
(b) Self-healing mechanism due to the severe lattice
distortion effect at 300°C – CCM: (AlCoCrCu0.5FeNi)3O4
F3- Smart Repairing (SR). Autonomous smart sensors in hydro pipelines assess defect behavior and plan maintenance. Advanced smart sensors activate chemical agents for structural filling, inhibiting crack propagation and restoring functionality (Fig. 5). Highperforming
metal alloys (e.g., Ni-based, Stainless Steel) ensure resistance to high pressures. External surface functionalization enhances tribological properties and corrosion resistance. A self-powered, removable, reusable monitoring system (e.g., thermocouples, pressure transducers)
supervises restoration quality and signals maintenance needs. A thin ceramic layer embeds the chemical agent, breaking during defects to repair pipes and restore operations.
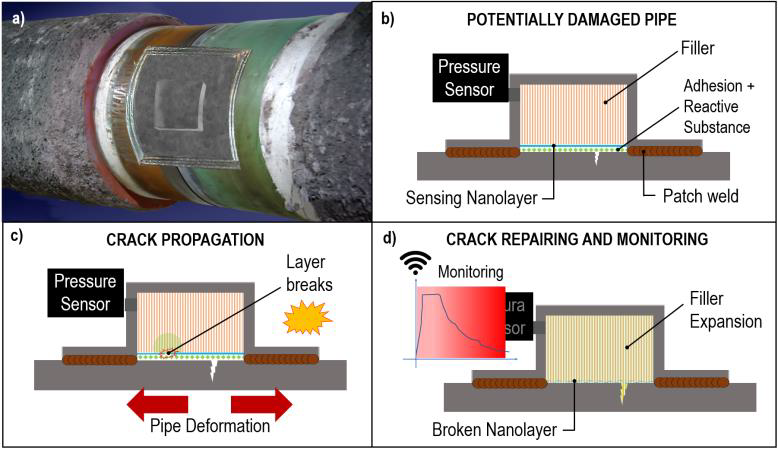
complex structure layout; (c) sensor activation; (d) crack repairing and monitoring
The objectives and ambitions
Project objectives are conceived and structured in order to cover the entire product lifecycle: from the design and engineering of multi-scale
smart functionalities into hybrid complex products, to the manufacturing of intricated and sophisticated solutions exhibiting macro graded
freeform shapes optimized by micro- and nanoscale structures, till the installation and operation of the realized smart product into industrially relevant working environments. WISE will integrate 5 processes into an all-in-one machine for the direct fabrication of macroscale products with smart functionalities at the nanoscale level (triggered biomolecules diffusion, selfhealing, and smart repairing).
WISE will achieve the following objectives:
O1 – Development and integration of product smart functionalities by implementing micro- and nano-scale structures into complex macro layer-wise components.
WISE will target O1 into three complex hybrid products:
a. Spinal cage prosthesis (MedTech sector) exhibiting graded mechanical and chemical properties developed to enable smart functionalities for improved healing treatments, ensuring increased biocompatibility (50% increase of implant lifetime) and enhanced osseointegration through TBioD-driven biomolecule transport (applied frequency from 3 Hz to 300 Hz). An ergonomic and lightweight design will reduce surgery duration up to 20% on average as of the result of the personalized geometric specs and the implementation of nanostructured surfaces will decrease the risk of implant rejection by 30%.
b. Turbine blade exhibiting self-healing mechanism (Aerospace sector) consisting of a CCM Functionally Graded Structure (dimensional accuracy < 0.05mm) ensuring 20%-60% enhanced resistance to oxide penetration and hot corrosion (e.g., molten salt corrosion) and an 20% increase of part lifespan under harsh working environment by exploiting unique core effects (such as high entropy, sluggish diffusion, severe lattice distortion, and cocktail effects) which increase part endurance to corrosive agents and activate self-healing mechanism at high working temperatures (up to 1500° C).
c. Healing patch for Smart Repairing (Oil & Gas sector) capable of monitoring in-situ the behaviour of structural cracks on pipelines operating in alkaline environments (water pH up to 8.4) by enabling autonomous repairing mechanisms (pipe diameter up to 1.5 m; crack depth range: 0.5 mm – 5 mm) when critical conditions occur (crack growth rate up to 1 mm/h) and tracking the status of the repair to schedule maintenance operations.
O2 – Product engineering for flexible and resilient production.
WISE will ensure a flexible, smart, and multi-scale product engineering based on the designed complexity and advanced functionalities required by the reference industrial scenarios, assisting product lifecycle from the design phase to the manufacturing, up to the operational environment (Design/Manufacturing-to-Lifevalue objective). Product engineering will be enabled by AI algorithms which, continuously updated by quality, process, and usage data (e.g., qualification reports, performance tests, maintenance operations, operational monitoring practices, online process data), will allow 50% faster response to design changes (rapidly setup the cycle for novel design) and reducing manufacturing lead time by up to 30%, (seamless synergy between product design and manufacturing requirements).
O3 – Manufacturing of high-quality hybrid products exhibiting complex geometries and smart functionalities enabled by multi-scale structure optimization.
The creation of intricate and sophisticated products that were previously unattainable by conventional fabrication methods will be enabled by layer-wise manufacturing, achieving freeform complex geometries enriched by functionalized 3-D micro- and nano-scale structures. Macro near-net-shaped components will show high-dimensional accuracy (< 0.05mm), high surface finishing (from 0.1 to 1 micron), and continuous variation of chemical, thermal, and mechanical properties by combining dissimilar materials (from CCA to ceramics to polymers). Exploiting the layer-wise manufacturing approach, the macro design will be functionalized by implementing micro- and nano-scale structures (resolution from 1 micron to 500 nm) enabling crack-free and high-density (>99.98%) hybrid components. WISE will target deposition building rates of 500 cm3/h and high material removal rates, covering a working area of 250mm x 250mm at 20 m/s.
O4 – Demonstrate the performance, design flexibility, and smart functionalities of the 3 complex hybrid products while in operation, showcasing the machine’s capabilities in the following areas:
a. MedTech: Spinal cage prosthesis will achieve a 15% increase in osseointegration and healing compared to current solutions, and a 25% reduction of fracture risk or implant rejection by increasing prosthesis lifespan by 20%-30%.
b. Aerospace: Smart CCMs turbine blade will achieve a 50% mechanical property recovery rate through self-healing by improving component lifespan by 20% and reducing inspection and maintenance operations by 10%. CCO coating will increase component resistance to oxide and hot corrosion by 30% compared to the current TBC solutions.
c. Oil & Gas: Smart sensors for gas pipelines with autonomous repairing mechanisms will reduce pipeline maintenance costs by 30% and downtime by over 60% by increasing component lifespan by 15%-20%. The sensor will enable more efficient pipeline maintenance planning (+30%) and an increased resistance to corrosive environments (+50% in salt water).
These ambitious objectives can only be achieved by integrating all the necessary processes into a single machine, ensuring that this integration is not an end in itself but leads to a synergistic reduction in costs and an increase in efficiency, necessary to exploit research in innovation. By consolidating multiple processes and leveraging advanced machine learning algorithms, the WISE machine will enable seamless interaction between various manufacturing techniques and material combinations, unlocking the full potential of advanced manufacturing for complex, multifunctional products. This holistic approach to process integration will not only streamline production but also foster innovation, as it overcomes the limitations of traditional manufacturing technologies and paves the way for new, ground-breaking applications across various industries. By accomplishing O1-O4 objectives, WISE will achieve the following outcomes:
a) Product Complexity: Through the integration of all necessary high-precision processes, WISE will facilitate the creation of intricate and sophisticated products that were previously unattainable.
b) Resource Efficiency: By consolidating multiple processes into a single machine and minimizing excessive movement, WISE aims to reduce process variability by a 20% reduction in changeover times and, as a result, decrease scraps and waste generated during lifecycle by at least 25%.
c) Flexibility: By employing AI algorithms, WISE will establish a highly adaptable combination of operation sequences, enabling a 50% faster response to design changes and reducing manufacturing lead time by up to 30%, allowing for seamless synergy between product design and manufacturing requirements.
The verification of WISE machine performance based on the achievement of O1-O4 objectives is central and transparent, divided into two milestones: (1) at month 18, all modules of the WISE prototype will be assessed to validate objectives O1 and O3 based on the performance of each module. This milestone will serve as a checkpoint to ensure that the individual components are functioning as intended and contribute to the overall effectiveness of the WISE machine. (2) at month 36, the integrated machine will be evaluated to confirm the fulfilment of objectives
O1 and O3, while the achievement of objectives O2 and O4 will be verified using data obtained from the full industrial demo. This two-step evaluation process ensures that the project remains on track and transparent, demonstrating the effectiveness and innovation of the WISE machine in delivering the desired results and advancing the state of the art in advanced manufacturing.
The verification of O1-O4 objectives will be carried out by comparing the current data provided by end users to the evidence of the impact that the achieved performance has on their products and businesses. This assessment will be conducted in a transparent manner and documented in a dedicated chapter of the exploitation plan, which will discuss this aspect in detail at the same two milestones mentioned above (month 18 and month 36). This approach ensures that the project’s advancements are not only technically sound but also directly contribute to tangible improvements in end-user applications and business outcomes, ultimately demonstrating the real-world effectiveness of the WISE machine.
The selection of the key processes to be integrated is driven by the product functionalities that need to be achieved.
Five high-precision process needs to be integrated.
P1 – Direct Energy Deposition (DED) will be used for the additive manufacturing of the macroscale semi-finished metal components. In the WISE project’s context, this process will be improved to overcome the current limitations of DED systems from the perspectives of material processability, process efficiency, and production of highquality functionally graded structures. A hybrid blue/IR laser source configuration will be designed and integrated into the machine, enabling the “right-the-firsttime” products of structural metal components consisting of both high heat absorption and high reflectivity metal alloys. In details, while metals such as Ni and Ti, with high heat absorption, can be effectively processed using standard IR laser (1064 nm), reflective metals like Cu and Au face limitations due to their poor absorption at this wavelength, resulting in processing defects like spattering and blowholes. However, to overcome this challenge, it has been found that blue laser radiation (450 nm) is a much better fit for these metals, with absorption rates of about 60% for gold and 45% for copper, as shown in Fig. A1. The high production flexibility provided by the coupling of the two sources in the same optical chain will be the turning key compared to the AM solutions currently available in the market, broadening the range of materials processable by a single system (i.e., the main limitation of AM systems), and enabling the cost-effective fabrication of high-quality composite materials engineered on the basis of advanced industrial applications (such as MedTech, Aerospace, Power generation). The DED module will be equipped with an innovative powder feeding system that will allow accurate on-the-fly control over metal alloy composition, enabling the production of high-density (greater than 99%) material gradient structures. To adapt the deposition rate to the desired resolution, the hybrid laser will be conceived with an adjustable spot diameter, considering timeoptimizations for parts that do not require high accuracy; as consequence, the powder feeder must adapt the powder flow without changing in alloys’ mixture.
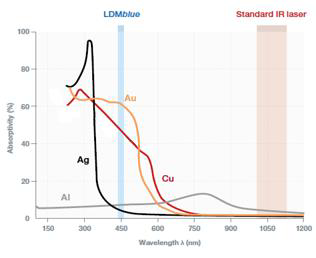
P2 – WISE will integrate the Direct Atomic Layer Processing (DALP), to perform deposition of the nanoscale metal and ceramic films on specific areas of the additive manufactured parts. It consists in an improved deposition of atomic layers compared to standard “in-chamber” methods in terms of process efficiency, reduction of waste and accuracy. The newly developed DALP technology (patented by ATLANT) overcomes the lack of area selectivity of ALD, by using a specialized deposition head (see Fig. A2) that enables emission of the precursors gases on a selective area, without spread in adjacent zones, resulting in an atomic deposition with micrometric spatial resolution in X and Y directions and a nanometric resolution in Z direction. The DALP ensures reduction in gas waste and costs. With DALP, unprecedented selective patterning is possible on a variety of surfaces, including flat, corrugated, and porous ones with application in different industrial sectors for the introduction of new smart properties, as shown in the definition of the 3 use cases in MedTech, Aerospace, and Power Generation sectors.
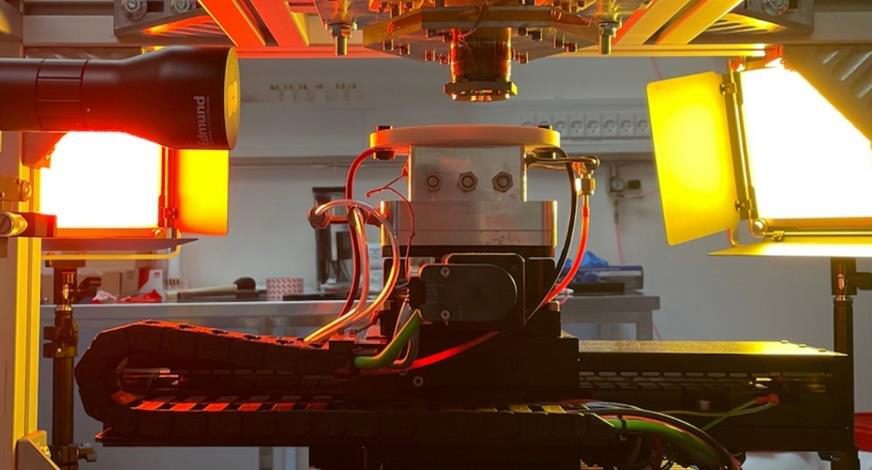
P3 – Femtosecond laser ablation (fs ablation) is a cutting-edge process chosen for enhancing the performance of components created through additive manufacturing. This technique relies on incredibly short pulses of laser energy, measured in femtoseconds (one quadrillionth of a second), to modify the morphology of metal surfaces. Unlike traditional methods, femtosecond laser ablation minimizes or carefully controls the amount of heat generated during the process, without thermal crack generation and changes in the mechanical properties of the processed components. The key outcome of this process is the creation of micro to nano-patterns on the metal surface, like grooves, bumps, pillars, and Laser-Induced Periodic Surface Structures (LIPSS), as shown in Fig. A3. These patterns have a profound impact on the material properties. The micro to nano-patterns created through femtosecond laser ablation significantly improve the material resistance to wear and tear. This means that components treated with this technique can withstand harsh conditions and maintain their performance over a longer
lifespan. Moreover, the modified surface properties can render materials self-cleaning and more resistant to fouling, which is the unwanted accumulation of substances like biofilms or contaminants on the surface. This property is particularly valuable in applications where maintaining a clean surface is critical. In the particular case of prosthetics, the modified surface properties facilitate better attachment to human tissue. This means that prosthetic devices can integrate more effectively with the body, improving their overall performance and comfort for the patient.
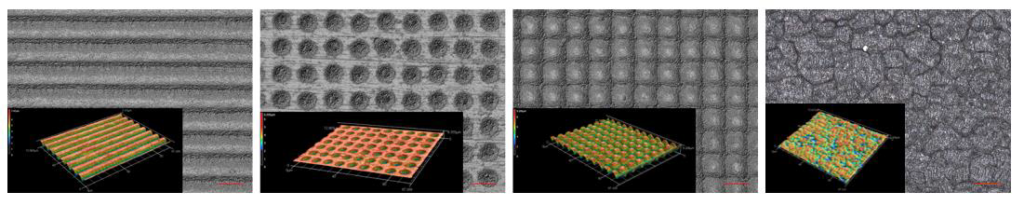
P4 – In order to create structured polymeric film on the functionalized surfaces of the manufactured parts the Two- Photon Polymerization (2PP) process will be exploited. The femtosecond laser pulses will initiate photopolymerization reactions of a liquid resin that will be liquid to build a nanoscale protective and isolating cover. The femtosecond laser ensures that this polymeric film is applied selectively, targeting only the areas that require it on the manufactured parts, providing precise protection to specific areas to enhance the durability and performance of the parts. The films created are incredibly thin, measuring on the nanoscale without interference with the overall dimensions or functionality of the components. Moreover, the functionalized surfaces benefit of isolation from external environment, with increased longevity.
P5 – Nanosecond laser polishing (ns polishing) of metal surfaces. The high surface roughness of metal surfaces on DED manufactured parts makes them unsuitable for direct deposition of nano-layers. To achieve the desired surface roughness of Ra less than 0.2 μm, a post-processing phase involving surface polishing is required. While the fs laser has applications in tasks demanding extreme precision and minimal heat effects (with ultrashort high intensity peak that results in precise of material and not in remelting) the nanosecond pulses guarantee the melting of small portions of material at a time to get a homogeneous surface (see Fig. A4). The CW laser would also be exploited for the heating
of the surface and consequent remelting, but the heat generated would propagate too deeply, causing changing on the microstructure of the
components, formation of thermal cracks and pores with weakening from the structural point of view.
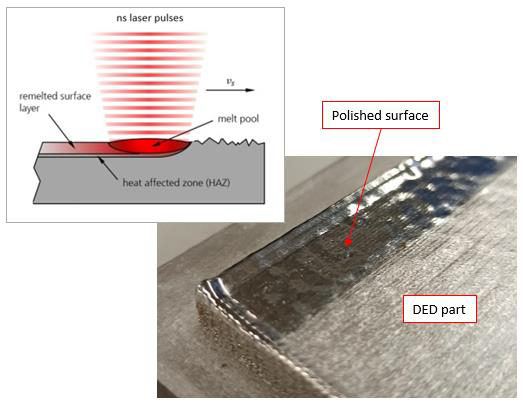
example of a polished surface.
WISE will overcome the current limitations in pulsed laser processes (P3, P4 & P5): low production efficiency for the cost of the equipment and difficulties in processing complex surface larger than the scanner’s field of view.
The fs ablation and 2PP suffer of high processing time: only small parts can be processed in reasonable time. To accelerate the process for industrial use, a high-power (300 W) high-repetition rate (40 MHz) femtosecond laser will be integrated and optimized for enabling the use of the Fast and Advanced Scanning and Tailoring (FAST) unit, which will be developed during the initial part of the WISE project. The FAST unit consists of an AI-powered scanner system and a DMD-based beam shaping feature, which acts as a spatial light modulator and allows for the
creation of custom beam shapes at superior speeds of up to 80 times faster than commercial scanning systems. It will ensure the processability of complex geometric features on an overall production surface of 250 x 250 mm in less than 30s, with a working time that is not dependent anymore on the scanner trajectories. Moreover, the scanner movements will be interpolated with the mechanical axes of the WISE machine to create an infinite field of view (IFOV) on all the machine’s total working volume to get increased productivity, improved accuracy, and greater flexibility in the design and production of complex parts, with consequent safe of time and energy consumption.
Moreover, to ensure flexible and resilient production, WISE will incorporate manufacturing and product knowledge in the AI-Aided Engineering Platform (AEP). The AEP will implement Virtual Self-Awareness for each manufactured product, thanks to a digital twin continuously updated with quality and usage data. This approach will allow for the monitoring and guarantee of product performance in their operational context, representing a critical challenge in their industrialization and dissemination.
Use cases
Three core use cases have been proposed by the consortium’s end users. These use cases will be developed along the project in terms of product (WP2), optimized with the AI Platform (WP3) and manufactured (WP9) with the WISE machine (WP 4 to 8) to demonstrate project technical impact and detail the corresponding business case (WP10).
Core use case UC1 – Spinal cage prosthesis (TBioD application).
Process description. The process starts with placing a metallic substrate and acquiring its position relative to the working table. Prosthesis functionalization is performed layer-wise based on product requirements, taking advantage of the AM processes in achieving freeform geometrical shapes enabling high product customization. First, the working volume is filled up with inert gas (e.g., Ar) to prevent both metal oxidation during laser-based processing and external contamination agents. Then the DED process is activated to manufacture a biocompatible layer core (e.g., Ti-based alloys, Co-Cr alloys) exhibiting macro-scale geometrical complexity based on the human body anatomy. Exploiting the layer-wise manufacturing approach and based on prosthesis complex design, surfaces responsible for bone tissue growth are
functionalized at micro- and nano-scale through ns and fs laser processing to promote biomolecule transport (TBioD) during the healing treatment (Fig. 6). Thereafter, a biocompatible photoreactive polymeric coating is applied using 2PP to ensure enhanced bioactive behaviour and boost bone fusion. Prosthesis areas not responsible for bone growth are coated with an ultra-thin layer (e.g., Ca10(PO4)6(OH)2, Ca3(PO4)2) via DALP. During product manufacturing, planned quality inspection stages are performed to check the quality of the functionalized layers against the part’s geometric complexity and process capabilities. Observed deviations from the optimum are removed by fs ablation and fixed. On the contrary, if the realized geometry meets the designed product requirements, the process sequence continues until the part is completed. The capacity consists of 1,000 functionalized spinal prostheses per year, with a worldwide surgery volume of about 500,000 annually.
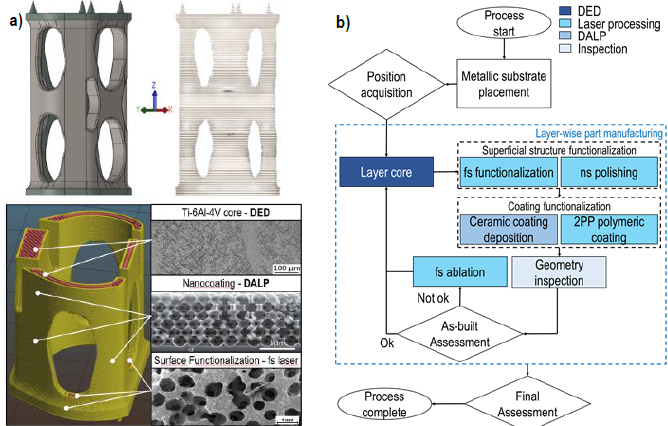
Core use case UC2 – Turbine blade (SH application).
Process description. The process starts with placing a metallic substrate and acquiring its position relative to the working table. To increase the final quality of the realized component (e.g., preventing material oxidation, oxide diffusion into the as-built component, and delamination), the process starts by filling the working volume with Ar. Then a core of Ni-based superalloy is realized by AM. The quality of the realized core is assessed by an online inspection stage. If deviations from the product requirements are detected, fs ablation is activated to heal the detected defects, otherwise, the CCAs’ functionally graded layer is applied on the Ni-based core by exploiting the DED capability of mixing dissimilar metal alloys or precursors to enable 3-D material gradients. IR laser is used for CCAs enriched in chemical elements with a high heat absorption coefficient (e.g., Ni, Cr, Co, Mn), while blue laser enables the production of CCAs with a high content of highly reflective precursors (e.g., Cu, Al). The layerwise manufacturing approach will allow the realization of graded complex structures enabling smart product functionalities, not achievable by conventional manufacturing techniques. Once the composite layer is realized, an inspection stage is performed to check as-built quality. If deposition defects are observed these are fixed, otherwise the external surface of the graded high entropy layer is coated by CCOs through DALP process. (Fig. 7). If the final inspection is successful, the process is complete; otherwise, coatings are removed and reapplied until requirements are fulfilled. The production scenario considers a capacity of 300 turbine blades per year, with a worldwide gas turbine blade volume of about 200,000 annually.
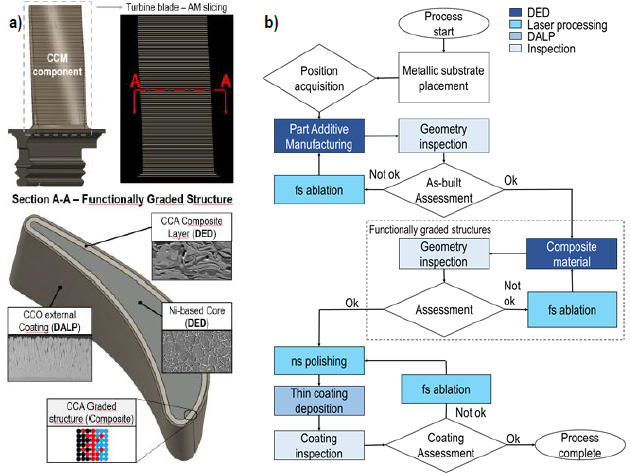
Core use case UC3 – Healing patch for Smart Repairing.
Process description. The process starts with placing a metallic substrate and acquiring its position relative to the working table. First, the cover of the smart sensor is created layer-wise using DED in an inert deposition environment by using metallic materials like Stainless Steel or Ni-based alloys. The advantage of using DED instead of casting or machining is that the AM process allows flexible product customization hardly achievable with conventional manufacturing processes, enabling sensor design more compliant with pipe and defect size, optimized based on
industrial needs. The quality of the as-built layers is assessed by an inspection stage. If the as-built component meets the product requirements, its surface is optimized by fs texturing and ns polishing to reduce surface roughness and to arrange the placement of both the reusable monitoring system and the chemical agent responsible for defect repair. A polymeric layer is applied to the external surface of the metallic cover through 2PP. Once the fs process is completed and the part meets the product requirements, both the chemical agent and the monitoring system are placed. Afterwards, a ceramic layer is applied through DALP to enclose the chemical agent into the smart sensor (Fig. 8). The final quality of the realized complex structure is assessed by an online inspection stage. If deviations from the optimum are observed, the ceramic coating is removed by fs ablation and a new DALP deposition is performed. Otherwise, the process is complete. The production capacity is 400 smart sensors per year.
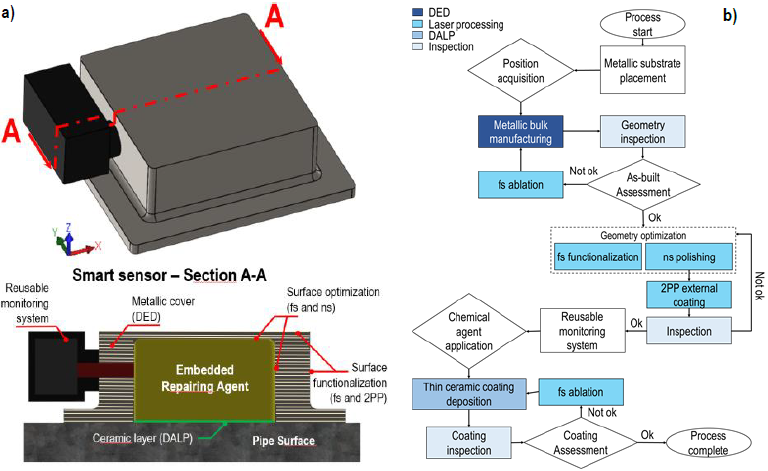
Compared to conventional process chains, WISE machine will enable:
- In light of the layer-wise process chain and in compliancy with the realization and integration of multiscale features (from nanostructures to freeform macro-components) into a single manufacturing system, the product functionalities can be only enabled by manufacturing of complex hybrid products in one machine (for every layer, it wouldn’t be viable to transfer the fixturing from one machine to another; it would violate the accuracy requirements).
- Reduced product lead time for the fabrication of complex-shaped hybrid products thanks to the integration of different manufacturing technologies into a single machine. Conventional fabrication processes usually require a sequence of independent manufacturing operations and systems to achieve geometrically complex functional components increasing product lead time and production costs related to machine setup, semifinished transportation, fixturing, tooling, consumables, component assembly.
- Improved manufacturing repeatability and efficiency by reducing manufacturing errors resulting from wrong product misalignment and positioning due to the sequential manufacturing process stage involved in conventional process chains.
- Increased automation and streamline process for high complex functional design exhibiting smart functionalities by enabling a layer-wise manufacturing approach.
- Increased product design flexibility and improved decision-making by reducing manufacturing and investment costs (system overhaul) resulting from product design changes.